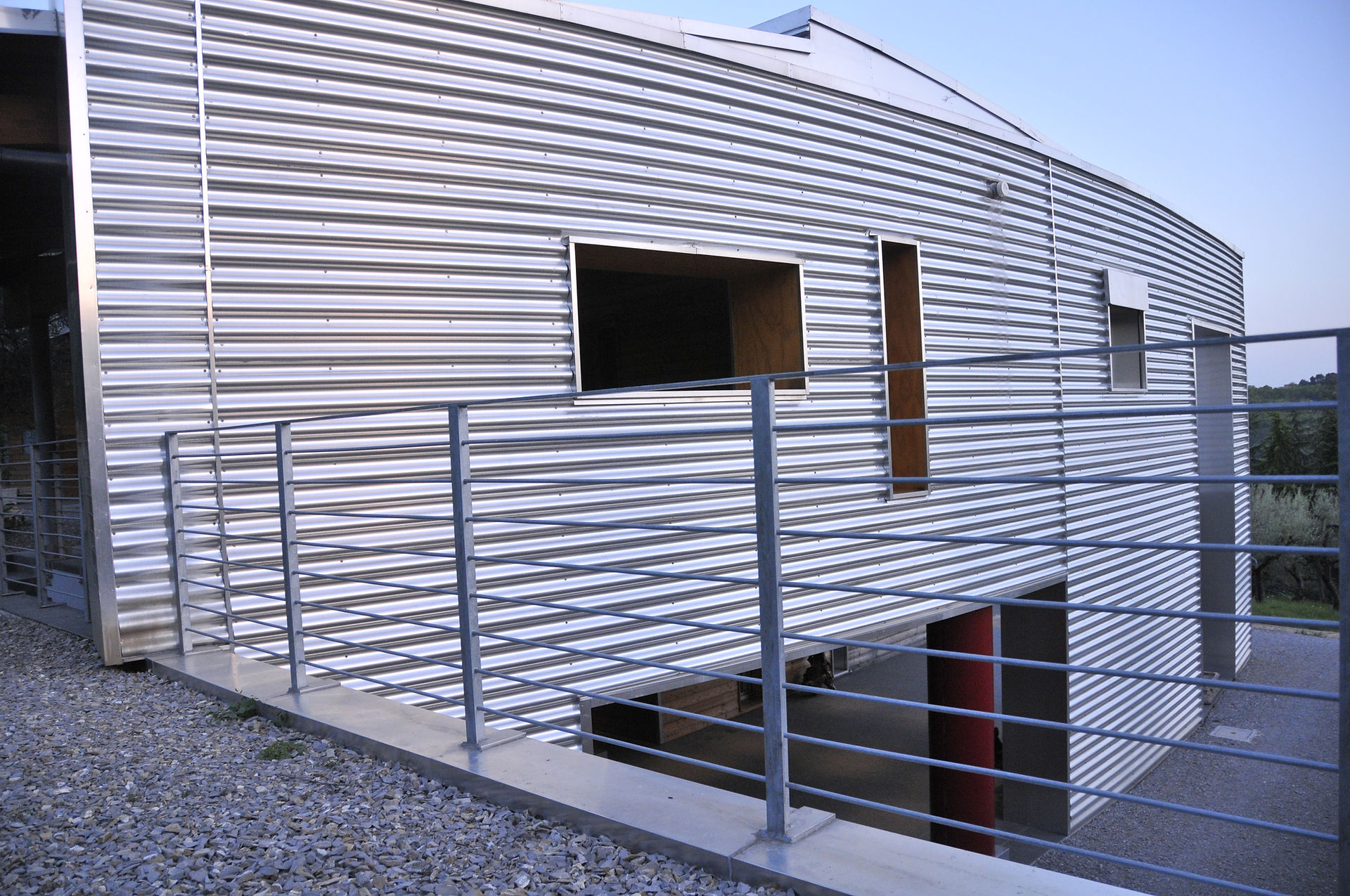
PERUGIA (IT)
PASSIVE HOUSE fontana
2008 - 2010
AN HOLISTIC APPROACH TO nZEB
The works concerned the construction of a residential complex with almost zero energy and environmental impact in Perugia, central Italy.
The project envisage a totally integrated approach in order to enhance indoor comfort and, at the same time, drastically reduce the environmental impact. Architectural design, structural efficiency, bioclimatic architecture integrated with the local environment, the use of low-impact construction techniques and materials, near-zero energy technical solutions and strategies, and the use of on-site renewable energy sources are all factors simultaneously involved in the design and construction concept.
Bioclimatic architecture.
The Umbrian climate is semi-continental and Mediterranean, strongly influenced by the presence of the Apennine mountain range, which interrupts the flow of air from the Adriatic Sea, mainly from the north-east. Outdoor temperatures are normally above 0° C (Richard Hyde et al., 2008). The municipality of Perugia benefits from a solar radiation of 1454 Kwh/(m2/year), a considerable amount of solar energy to be exploited in winter through passive solar heating, and to be controlled in summer to reduce the risk of overheating. This has been achieved through specific technical and design strategies and solutions: east-west orientation of the building with large openings to the south to maximise winter solar gains; large porch on the south front to protect the windows during the summer; distribution of day functions along the south front (daytime solar heating and direct light in winter) and night functions along the north front (opaque and transparent envelope with higher thermal insulating capacities and smaller window sizes); external motorised blinds on all openings on the south and west fronts; dark and rough external paving (in recycled artificial stone) to reduce solar radiation reflection phenomena on the south and west fronts; ventilated aluminium and wood façades, reflective aluminium roof with double ventilation, to reduce overheating in summer and facilitate transpiration in winter; arrangement of window openings to allow natural night ventilation between the south and north fronts. The efficacy of the bioclimatic architecture measures applied is confirmed by the survey data of the internal temperatures and relative energy consumption measured during two winter days in the same year (2012), one sunny and one cloudy: thanks to the sun, energy consumption was 40% lower despite the external temperature being lower.
Technical solutions and strategies for nearly zero energy consumption.
Unit 1 of the nZEB residential complex in Perugia Fontana, is a certified passive building, n° 2929 of the Passive House International Database at the PassivHaus Institut in Darmastd (DE): in a passive building thermal comfort (UNI 2006 - ISO 7730) can only be achieved by post-heating or post-cooling the fresh air mass that is required to meet indoor air quality requirements (Feist, 2015). Passive House is therefore an integrated concept: in a perfectly insulated and airtight building, in which the use of an efficient mechanical ventilation system is essential, the ventilation system itself can be used for heating and cooling without the need for additional traditional systems (Feist 2015). Furthermore, in buildings with natural thermo-hygrometric comfort conditions, it is essential to integrate all energy efficiency factors in order to minimise energy use (Boeri and Longo, 2011). The factors on which integrated action was taken can be summarised as follows: thermo-hygrometric performance of the envelope; air tightness; minimisation of thermal bridges; ventilation system; implementation of the use of RES on site.
Thermo-hygrometric performance of the opaque envelope.
The thermo-hygrometric performance of the opaque envelope has been designed using solutions capable of guaranteeing an adequate balance between thermal insulation in winter and thermal inertia in summer: light walls and roofing in wood and wood fibre (specific heat 2,100 J/(Kg K); density 140/150 Kg/m3), allow for a high level of phase shifting and damping of the thermal wave and produce a strong attenuation of the peak of incoming summer thermal energy and a time lag of over 20 hours. These performances are comparable with those of a traditional stone envelope but, on the contrary, with a much higher thermal insulation capacity (wood fibre conductivity 0.038 W/(m K); stone thermal conductivity about 1.5/2 W/(m K). The density of the insulating material was differentiated in relation to the solar exposure of the part of the envelope concerned (higher density for southern, western and roof exposure, as described above, lower for the other parts). The floor of the ground floor was designed with a higher thermal conductivity than the rest of the envelope, U = 0.151 W/(m2 K), in order to reduce the cooling demand by facilitating the heat transfer to the ground during the summer period, but without compromising the relative winter performance. The simulations carried out with dedicated software, had shown a balanced situation between winter and summer performance, then confirmed by the measured performance, despite the presence of large glass openings on the south front. Thermal masses were also added, such as the concrete screed of the ground floor floor and the vertical AC bracing baffles, with the aim of acting as thermal heat accumulators during summer days. In addition, in order to minimise internal summer loads, a by-pass was used for evening and summer free cooling. The opaque envelope has been designed to be permeable to water vapour, according to UNI EN ISO 13788: this standard introduced a different approach to vapour diffusion management from that traditionally used, accepting the idea that vapour can migrate through the structures of a building and, albeit in a small part, condense inside and then evaporate in the following summer season. This philosophy has been fully embraced by the project for which (controlled) vapour permeability of the envelope, together with the adoption of vapour barriers and a mechanical ventilation system, is the best way to avoid the risk of interstitial and surface condensation in wood fibre insulated envelopes in temperate climates.
Thermo-hygrometric performance of the transparent envelope.
The transparent envelope has been designed using mixed wood (internal) and aluminium (external) frames insulated with expanded polystyrene, which also acts as a thermal break. The glazing is of the low-emissive two-chamber (triple glazing) type, insulated with Krypton gas for northern exposure (Ug=0.48 W/m²K), and Argon for southern, western and eastern exposure (Ug=0.71 W/m2 K). The U-values of the external windows and doors were varied in relation to the exposure, as shown in the table below. A stainless steel channel connects the frame to the glazing, guaranteeing a Ψ = 0.051 W/(m K) value at the connection, while a self-expanding vapour barrier tape connects the frame to the wooden structure of the external curtain walling, ensuring a perfect airtightness. In order to minimise the thermal bridge between the frame of the window and the wall, an external coat of wood fibre was laid along the perimeter of the connection between the two components, with an excellent Ψ = 0.038 W/(m K) value.
The ventilation system.
The ventilation system continuously takes exhaust air from the kitchen, bathrooms and rooms with high humidity and pollutants, while supplying fresh outside air to the living room and bedrooms. The system is equipped with an exchanger that recovers 85.8% of the heat present in the exhausted air at the outlet, transferring it to the fresh incoming air without the two flows mixing. With such a low level of permeability of the envelope and such a high percentage of air heat recovery, energy losses through ventilation become insignificant. Furthermore, thanks to the heat recovery system, the temperature of the fresh air is close to that of the indoor air, so that the fresh air is no longer 'cold' air. This, together with the excellent thermal insulation of the opaque and transparent envelope, drastically reduces the thermal and cooling loads and reduces the size of the systems and their energy consumption: it becomes possible to heat and cool using only fresh air. And because it is fresh air, not recirculated air, the amount of fresh air is limited, which reduces the risk of the air becoming excessively dry. However, considering that the air temperature cannot be increased too much, heating (and cooling) by ventilation alone can only be successfully used in buildings with minimal energy requirements such as NZEBs and Passive Houses (Feist, 2003). A temperature sensor determines if and when to stop heat recovery from the outgoing air, or to activate the by-pass to expel air without recovery (evening or summer free cooling). To increase the passive performance of the ventilation system, an underground heat exchanger (Provençal or Canadian well) has been built to pre-heat and pre-cool the incoming fresh air.
Implementation of the use of renewable energy sources (RES).
As Italy is a country that imports most of its energy, it is of fundamental importance for any new building to increase the use of renewable energy in order to strongly encourage any energy independence initiative (European Union, 2016). But it is equally fundamental to develop solutions for which energy savings are achieved through an integrated design that combines passive building solutions with simple renewable energy production systems such as solar, without resorting to high-tech or overly innovative technological solutions (Ferrante and Cascella, 2011). Three types of renewable energy sources have been foreseen in the integrated design concept: low-enthalpy geothermal; solar (thermal for DHW production and electricity); and aerothermal (heat pump). The geothermal renewable resource is used for passive pre-heating (in winter) and pre-cooling (in summer) of the fresh air: before it is introduced into the building through the ventilation system, the air is forced to pass into a Provencal Well (underground heat exchanger), a simple system of food-grade plastic ducts buried at a depth that allows the exchange of heat or coolness with the ground.
The solar resource for the production of electrical energy consists of a system of photovoltaic panels fully integrated into the metal roof of the building, CIGS thin film type with a surface area of about 40 m², connected to the grid which produces a peak energy of about 6.4 kWe. The solar resource for the production of DHW is a system of thermal panels of the "drain back" type, with a surface of about 8 m², which heats more than 80% of the DHW needed during the year. Solar DHW is also used for washing machines and dishwashers through special appliances. The aerothermal renewable resource, in accordance with point 31 of Directive 2009/28/EC, is a reversible heat pump with a power of only 6 kW which, through a battery, heats or cools the air after geothermal treatment (and/or after heat recovery in winter) so as to guarantee a minimum indoor temperature of 20°C in winter and a maximum of 26°C in summer with almost zero energy consumption.
Use of natural and/or low-impact techniques and materials.
In 2007, Sartori and Hestnes pointed out that designing buildings with low energy consumption reduces the overall energy impact during their life cycle, but can result in an increase in embodied energy. Embodied energy in buildings is continuously increasing due to increased performance during the operational phase in the direction of near-zero energy consumption. In an NZEB, therefore, there is a real risk of shifting impacts from the operational phase to the construction and end-of-life phases (Asdrubali et al.,2013)". However, through the application of LCA-type methods, it is possible to improve the quality of construction techniques and materials, heating and cooling systems and energy production from RES, achieving a significant reduction in the overall environmental impact of the building at each stage.
It is therefore strategic to carry out a careful analysis of the overall environmental impact of each new building, starting from the design phase, especially for nZEB buildings, in order to avoid the transfer of environmental loads from one phase of the building's life to another. The NZEB low-impact residential project in Perugia included the use of the LCA method right from the design phase in order to assess and minimise the overall environmental impact of the project. The analysis of the LCA cycle with contributions from electrical RES from PV, carried out by comparing the NZEB with an ordinary building, shows how the global impacts generated by construction, maintenance, management and decommissioning are significantly lower in the building with almost zero energy consumption, and are cancelled by a contribution of RES of about 10 kWh/m². The main strategies adopted in this respect are the following: reduction of energy consumption; production of energy from RES on site; prevalent use of dry building systems designed for selective deconstruction; use of easy-to-use technologies and materials with reduced maintenance needs; use of natural or EPD certified materials (UNI 2001 - 14024 and UNI 2010 - 14025); use of recovered/reused and/or recycled/recyclable materials; use of materials from renewable resources; drastic reduction in the end-of-life of Construction and Demolition Waste, below 70% of the total weight as foreseen by 2020 by Directive 2008/98/EC.